Water Electrolysis for Hydrogen Production Facilitates Decarbonization
The thermal processing industry is a good example of how the on-site production of hydrogen by water electrolysis can be beneficial for many of its processes and for reducing the CO2 of its plants. In today’s Technical Tuesday, David Wolff, industrial sales director at Nel Hydrogen, discusses how, from plasma spray to metal AM binder jet to annealing at rolling mills, industries across medical, automotive, and beyond are looking to water electrolysis for hydrogen production.
This informative piece was first released in Heat Treat Today’s December 2024 Medical & Energy Heat Treat print edition.
Hydrogen atmospheres are widely used in high temperature thermal processing, including annealing, brazing, PM, MIM, and binder jet AM sintering, metal-to-glass sealing, and related processes such as thermal spray. Hydrogen helps heat treaters achieve acceptable product characteristics. It’s used as a very powerful reducing agent, and it actively cleans surfaces as compared to inert gas atmospheres which only displace oxygen.
Relative to hydrogen’s use in helping plants decarbonize, it’s a fact that major OEMs buying heat treating services and heat treated products are demanding that their suppliers report their decarbonization progress. To meet the needs, hydrogen generation is becoming ever more compelling to heat treaters to ensure hydrogen for atmosphere needs inside the plant, and to help minimize their carbon footprint.
The Clean Energy Supply Conundrum
Most U.S. heat treating facilities get their atmosphere components delivered by truck. The truck emits CO2 and the hydrogen on that truck is likely “gray” hydrogen made from natural gas. Hence, the carbon footprint from their hydrogen use is notable. Importantly, the electricity grid operators are actively seeking ways to enhance the business success of providers of low carbon electricity. The key issue with those providers — solar, wind, hydro, and nuclear — is that they cannot easily follow the ups and downs of demand. Instead, consumers get electricity from those resources when the wind is blowing, the sun is shining, or the river is high. In the case of nuclear plants, they preferentially run at near fixed output, day and night. They run continuously regardless of demand. As the grid demand is very low at night, they get very low prices for the electricity they generate. They only make money for 12 or so hours a day. That’s why a lot of nuclear plants are threatening shutting down for economic reasons.
Taking Advantage of Low Demand Period Energy Prices for Use During High Demand Hours
Consider this scenario: What if a client with electrolysis capacity to produce hydrogen, such as a heat treater, could buy electricity at lower nighttime prices to make the hydrogen it needs during the day shift for its various processes, perhaps even heating their furnaces? The clean energy provider would be pleased to have more income during its low demand, low price times. The heat treat plant is happy saving money buying decarbonized electricity at low demand prices to make clean hydrogen for its various thermal processes and to operate its furnaces. And, the heat treat company’s OEM clients demanding decarbonization are satisfied, too.
How To Get Started
The scenario described above is a practical and real one for the heat treat industry today. Nel Hydrogen recommends that a heat treat company begin with a plan. That plan may comprise several phases. It’s important to seek out a knowledgeable hydrogen partner in this endeavor to specify exactly what’s needed. For heat treat applications, users generally would want compact equipment, extreme hydrogen purity, load following, near-instant on and instant off, and sufficient hydrogen pressure that make it flexibly suited for a variety of thermal processes, and for hydrogen storage addition at a later time if desired.
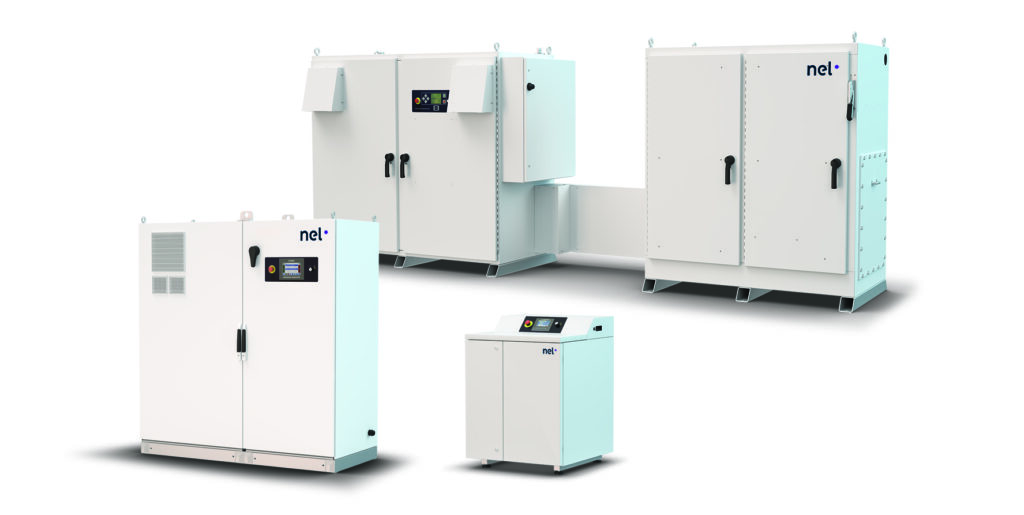
Both batch and continuous processes can be served. Batch processes may benefit from a small amount of surge storage at the outset. By combining on-site hydrogen generation with a small amount of in process hydrogen surge storage if needed, on-site hydrogen generation can be used to meet the needs of batch processes such as batch furnaces and thermal spray. By carefully choosing generation rate and pressure, and surge storage vessel volume and pressure capacity, the combination of generation with surge storage can provide maximum process flexibility while minimizing the amount of hydrogen actually stored.
The presence of a small amount of hydrogen surge storage also protects clients’ parts in case of an electric interruption that stops hydrogen production. The surge storage hydrogen can protect the parts while they cool under a reducing atmosphere.
In practice, specific client priorities such as minimum hydrogen storage, or lowest system capital cost, or highest degree of expandability, or least amount of space occupied, can be met by choosing the specific hydrogen generator capacity and surge storage system employed for any particular production challenge.
Examples of Thermal Processors Producing Hydrogen On Site with Water Electrolysis
Decarbonization will be a near-future requirement as part of the global effort to evolve towards a cleaner, greener world. On-site hydrogen generation in industry makes great sense to align with those initiatives. Right now, the thermal processing industry is experiencing the benefits of producing hydrogen on site for its production processes, and the decarbonization demand will be easier to accommodate with that infrastructure in place.
Here are a few examples of companies performing a variety of thermal processes that have made the decision to use water electrolysis to produce hydrogen on site:
Plasma Spray of Cast Iron Cylinder Liners
One of the most compelling examples has been implemented by two different U.S. automakers to accommodate the increasing use of low-weight aluminum engine blocks in today’s high efficiency vehicles. Aluminum blocks must have a cast iron lining on the inside of the cylinder bore to maximize the durability of the engine. (Older readers may recall the notorious Chevy Vega that used an aluminum engine without a cast iron liner. The author’s wife had one Vega which burned through three engines!)
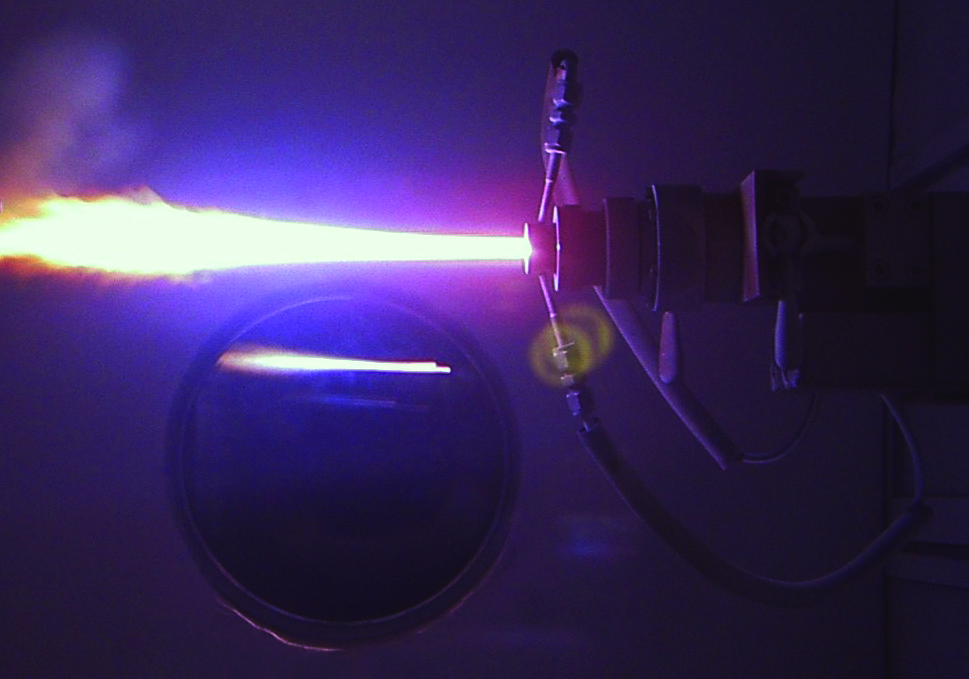
The traditional approach to provide a cast iron liner was to drive a sleeve into the aluminum engine block. However, a new technology has been commercialized by which the cast iron liner is spray-applied using a plasma torch. The torch uses hydrogen and argon gases to add energy and maintain the necessary low oxygen atmosphere. The plasma spray was a new addition to engine production facilities that had not previously been equipped with hydrogen supply and thus elected to generate their own to minimize delivered hydrogen and avoid the need for hydrogen inventory and extensive supply piping.
The electrolyzers recommended for plasma spray applications are compact and produce high purity hydrogen of better than UHP grade at 200+ psig pressure, with less hydrogen stored than would fill a party balloon bouquet. About the size of a washing machine or refrigerator, depending on the model, each unit is low maintenance, compact, quiet, and can be installed nearly anywhere in a facility.
Metal Additive Manufacturing (AM) Binder Jet
One of the most exciting approaches to metal AM is the technology called binder jet, which creates a near net shape part using polymer and wax binders to adhere metal powders. After the part is formed, the binders are chemically or thermally removed. Then the part is sintered to attain near net shape and full part density. Hydrogen is required for the sintering atmosphere to prevent oxidation of the part during the sintering process. Binder jet technology promises to provide for mass production of individually customized parts at high production rates and consequently lower costs than parts produced individually.
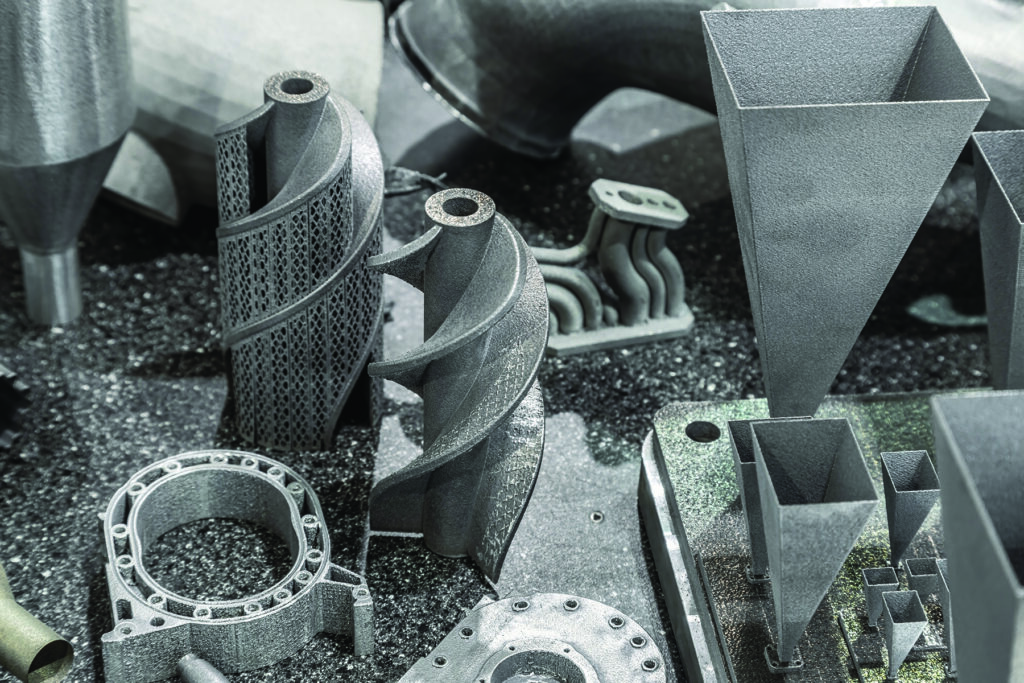
Many new metal AM production facilities are being established in factories that are not already equipped for the delivery, storage, and internal piping/distribution of hydrogen. As such, many have chosen instead to use zero inventory hydrogen made on site to minimize infrastructure investments. Electrolyzers for small-scale applications requiring up to 230 scf/hr of hydrogen gas at 99.999+ % purity are advised for metal AM. About the size of a large refrigerator, the units require minimal facility floor space, are easy to maintain, and can be installed in any non-classified space. Applications for AM include medical, electronics, industrial, and automotive components.
Annealing at Rolling Mills
Plate and strip metal are processed in rolling mills where the thickness of the metal is reduced by alternating “cold” rolling steps followed by intermediary hot annealing steps. Cold rolling makes the metal more brittle, so it is necessary to have an annealing step following each rolling step. The metal is alternately thinned and then softened for what could be several iterations. Hydrogen is required for the annealing steps to maintain metal surface quality while heated. Because of the periodic market disruptions in delivered hydrogen from plant outages or trucking interruptions, several rolling mills have chosen to generate hydrogen on site to augment or entirely replace their delivered hydrogen supply. The benefits that the plants experience are primarily focused on supply reliability. Of course, they are also eliminating the carbon footprint associated with truck delivery. In this case, the carbon footprint of the generated hydrogen is determined by the particular electricity generating mix that serves the plant site.
Most often at rolling mills, electrolyzers that produce up to 1,140 scf of hydrogen gas at 99.999+ % purity are best suited for the hydrogen requirement. These units replace the need for hydrogen tube trailers or liquid hydrogen storage. They can be installed in the mill or can be containerized outdoors, offering flexible siting and reduced operational safety risks compared to delivered hydrogen.

On Track Towards Decarbonization
Described in the examples above, once the means to generate hydrogen is chosen at a thermal processing facility, the company can move further along the decarbonization journey. This may be to apply a strategy as outlined in the electricity scenario whereby the company takes advantage of low demand rates or institutes an alternative creative idea. Certainly, as more and more clients demand proof that suppliers are reducing their carbon footprint, more strategies will be developed and implemented to serve the thermal processing industry. Simply generating hydrogen on site removes the trucking emissions factor and is a beneficial and practical starting point.
About the Author:

Eastern Regional Sales Manager
Nel Hydrogen
David Wolff has 45 years of project engineering, industrial gas generation and application engineering, marketing and sales experience. He has been at Nel Hydrogen for over 25 years as a sales and marketing leader for hydrogen generation technologies.
For more information: Nel Hydrogen at sales@nelhydrogen.com.
Find heat treating products and services when you search on Heat Treat Buyers Guide.Com
Water Electrolysis for Hydrogen Production Facilitates Decarbonization Read More »