Evolving Efficiency: Advantages of Multi-Chamber Isolated Heat Vacuum Furnaces
Adapting to new processing demands puts traditional equipment to the test. Can single-chamber solutions keep up, or will applications require different equipment options for efficient processing? In today’s Technical Tuesday, Bryan Stern, product development manager at Gasbarre Thermal Processing Systems, addresses the advantages multi-chamber isolated heat vacuum furnaces bring to the floor.
This informative piece was first released in Heat Treat Today’s November 2024 Vacuum print edition.
Do You Hear That? It’s the Sound of Change . . .
In the evolving landscape of vacuum heat treatment, single-chamber batch furnaces have long been the cornerstone of material processing. However, with more traditional processes shifting to vacuum, rising energy costs, and increasing environmental pressure, the disadvantages of that approach are emphasized, enhancing the appeal of alternative technologies. Multi-chamber vacuum equipment, while not new to the industry, offers significant solutions to inefficiencies and challenges faced by single-chamber systems. With advances in technology, improved operational planning, and an increasingly competitive market, multi-chamber isolated heat furnaces are becoming a more viable choice.
What Is an Isolated Heat Vacuum Furnace?
An isolated heat vacuum furnace keeps the heat chamber separate from the ambient atmosphere throughout the process, including loading and unloading. This allows the heated zone to maintain a stable temperature and vacuum between cycles, unlike single-chamber furnaces, which must heat up and cool down for each new load. Key components of this furnace type include an additional evacuation chamber, a dynamic sealing door, and a mechanism for moving the workload between chambers. While multi-chamber isolated heat furnaces may be batch or continuous, the above features fundamentally distinguish them from single-chamber batch equipment. This difference is more than just a technical nuance; it has profound implications for operations and efficiency.
The widespread use of single-chamber vacuum furnaces has significantly shaped the design and operation of vacuum furnaces today. But it is important to remember some of the challenges to this approach that we often take for granted.
Energy Efficiency Has Entered the Chat
Single-Chamber Challenge
In single-chamber systems, the entire furnace must go through a full cycle of loading, evacuation, ramping, soaking, cooling, and unloading for every batch of parts. This adds significant “dead time” on either side of the thermal process. In addition to pump-down time, ramping from room temperature typically adds 1–2 hours to the cycle time before soaking which creates a barrier to throughput. Another drawback is that the energy required to heat the furnace is thrown away after every cycle. Due to the high thermal capacity of materials like graphite and molybdenum, this is not inconsequential. With 100% thermal efficiency defined as only consuming the energy required to heat the work and fixturing, single-chamber batch furnaces typically operate in a thermal efficiency range of around 30%–50%.
Isolated Heat Advantage
In an isolated heat furnace, the work zone remains at temperature and the energy required to heat the furnace is not thrown away. Additionally, the introduction of work to a preheated work zone allows the load to be heated more quickly, reducing the time required to achieve temperature and reducing holding losses. While multi-chamber batch furnaces experience some savings, they still consume excess energy since the heat cage is empty during unloading, loading, and evacuation. Continuous configurations, however, see significant improvement with only holding losses and the energy required to heat the work and fixturing being consumed. These advantages mean that continuous furnaces typically operate in a thermal efficiency range of 45%–65%. The result is a 15%–35% energy efficiency improvement over the majority of existing equipment.
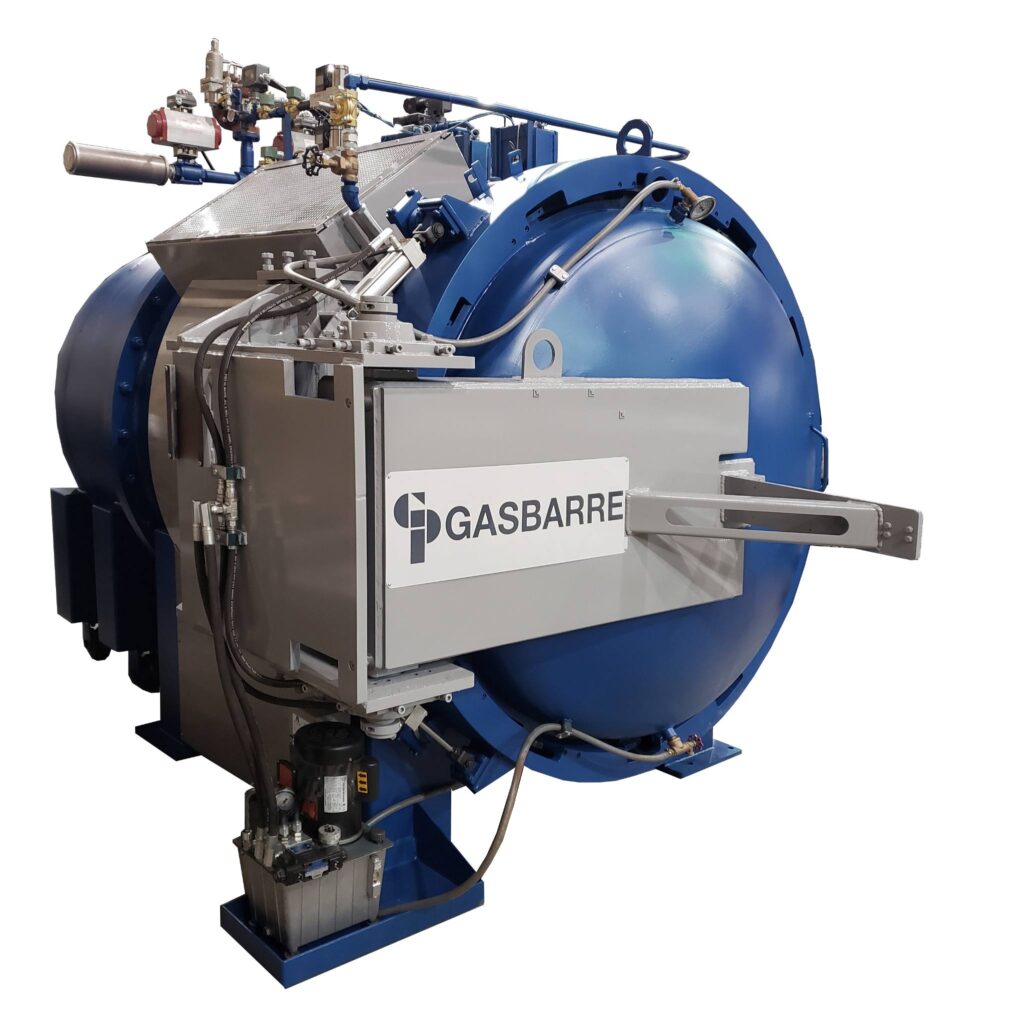
Source: Gasbarre Thermal Processing Systems
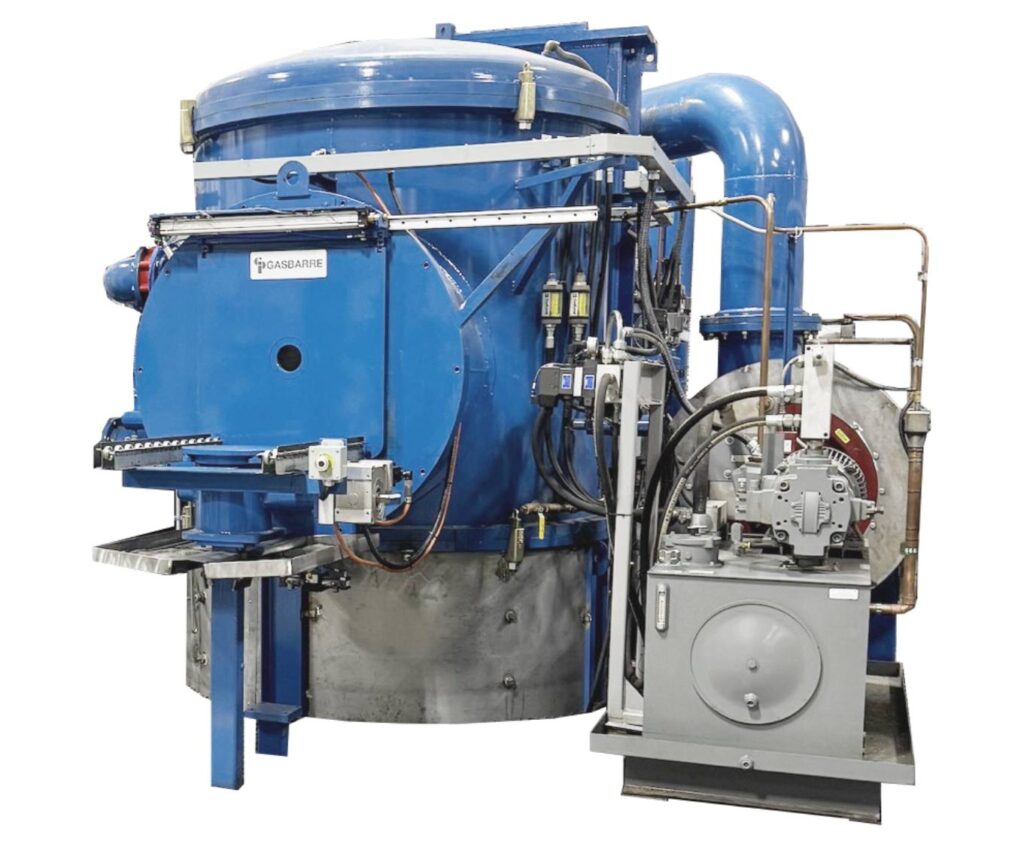
Source: Gasbarre Thermal Processing Systems
Design Optimization: Do I Detect Some Tension?
Single-Chamber Challenge
The tension of designing a single-chamber furnace to handle both heating and cooling in the same space presents substantial challenges. Insulation pack thickness is often limited to balance the need for quick pump-down. Gas nozzle penetrations through the insulation pack create direct radiation losses. This erodes thermal efficiency, adds thermal mass, and restricts gas flow during cooling. These conflicting design priorities often lead to unsatisfactory compromises and fluctuating designs. Between the additional energy to heat and cool and increase power demand at temperature, there are a lot of energy savings being left on the table.
Isolated Heat Advantage
Because the heating and cooling take place in separate locations, multi-chamber isolated heat equipment benefits from the ability to have dedicated designs tailored at each work position. More insulation can be used as conditioning time is not a significant consideration. Additionally, the insulation can be designed without penetrations, further reducing losses. Moving the work to a dedicated cooling position removes restrictions to gas flow and allows the work to radiate directly to the cold wall. This is especially beneficial at the beginning of a quench when the work is at high temperature. This can allow cooling rates to be achieved with lower quench pressures and smaller quench motors.
Thermal Cycling: Here We Go Again . . .
Single-Chamber Challenge
A single-chamber furnace must be built to endure extreme thermal cycling again . . . and again. This requires detailed design consideration to account for thermal shock, expansion, ratcheting, creep, and low-grade oxidation — all of which contribute to maintenance and replacement cost for expensive, long lead refractory components.
Isolated Heat Advantage
Since the heated portion of the furnace remains at stable temperature and vacuum, internal components are not subject to the same destructive forces. An isolated heat cage can remain in service much longer before requiring service or replacement. It also decreases the likelihood of sudden and unexpected equipment failure. Increasing the lifespan of the most expensive consumable assembly in the furnace is an incredibly valuable advantage that is frequently overlooked.
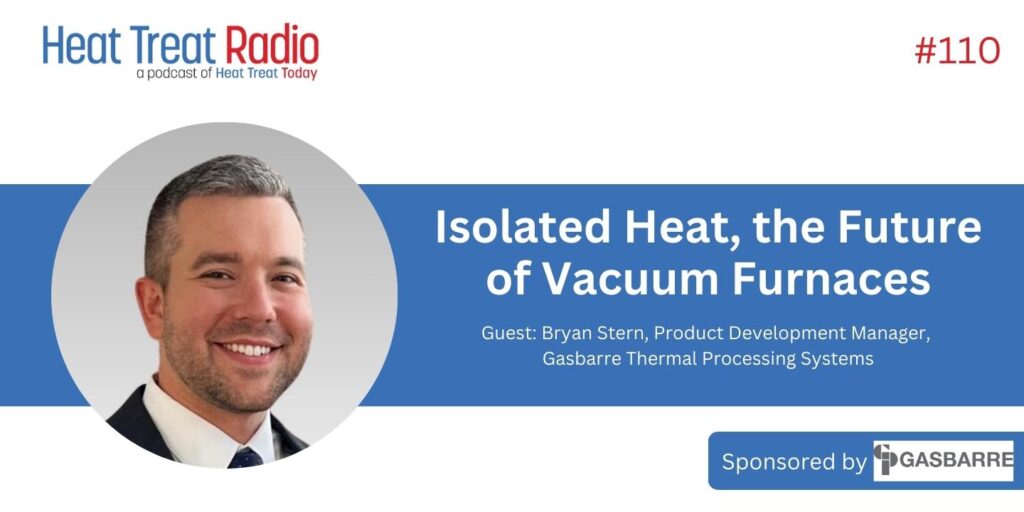
Throughput and Load Size: Can They Help?
Single-Chamber Challenge
Single-chamber batch vacuum processing is notorious for the long cycle times and resulting limited throughput. One way to reduce the costs of the wasted energy and dead time is to increase the load size to distribute the cost over more work. While this can increase capacity and reduce the cost per part, it is counterproductive to many objectives of the heat treating process. As the load size increases, it becomes more difficult to maintain thermal and process uniformity across parts at the surface versus the center of the load. This is especially problematic for densely packed loads. Loads take longer to soak out to a uniform temperature, extending cycle times. Similarly, it is difficult to achieve rapid and uniform cooling rates which can lead to higher quench pressures, larger cooling motors, or underutilizing the work envelope.
Isolated Heat Advantage
While multi-chamber batch isolated heat furnaces experience many of the other advantages discussed in this article, throughput is where continuous configurations really shine. Because separate loads are being processed simultaneously, similar or greater throughputs can be achieved with much smaller load sizes. For instance, a process with a two-hour soak would typically require around a five-hour total cycle time in a single-chamber furnace. That same process could be segmented in a continuous furnace indexing loads in as little as 15 minutes, depending on the configuration of the equipment (see Figure 3). With a throughput ratio of 20:1, each load would only need to be 1/20th of the batch load to achieve the same throughput. With these mechanics, it quickly becomes apparent how continuous processing is capable of achieving much greater throughput while benefiting from the uniformity of smaller load sizes as well as the other advantages discussed.
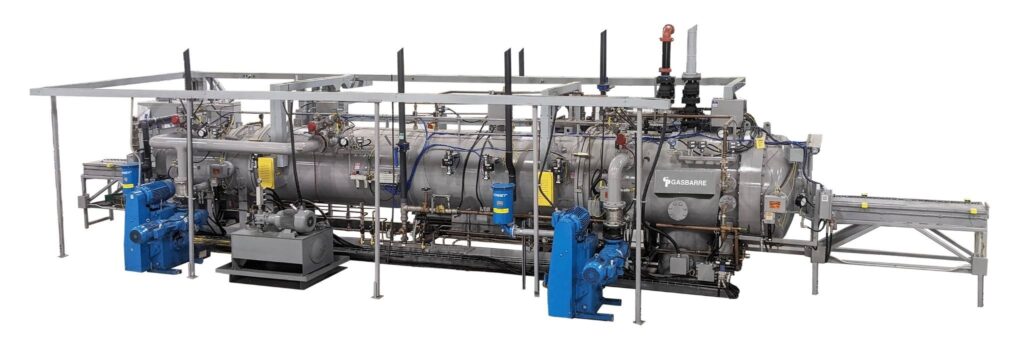
Source: Gasbarre Thermal Processing Systems
Scalability: And Another and Another . . .
Single-Chamber Challenge
Increasing the capacity of a single-chamber production line necessitates adding additional discrete furnaces. This means that all of the equipment systems are duplicated. Each furnace means another chamber, pumping system, manifolds, quench motor, VFD, control cabinet, certifications, instrument calibrations, etc. There really is no economy of scale available to help facilitate high volume production.
Isolated Heat Advantage
For most processes, increasing the capacity of a continuous multi-chamber furnace only requires adding additional heated work positions to shorten the index rate. All other auxiliary equipment and infrastructure can serve double-duty, and redundant systems and maintenance are avoided. This applies the cost directly to the necessary equipment (heat cage, elements, power supply, etc.). The resulting economy of scale often makes continuous equipment a far greater value proposition for high-volume applications that would otherwise require multiple furnaces.
Vacuum Performance: Don’t Reduce Me Like That!
Single-Chamber Challenge
Because single-chamber batch furnaces are exposed to air and humidity between each cycle, they require a higher vacuum (i.e., lower pressure) to achieve the purity required for a given process. This is because even though the furnace is evacuated to a low pressure, the remaining atmosphere is still primarily comprised of oxidizers in the form of residual air and water molecules desorbing from the internal surfaces of the furnace. Achieving the high vacuum levels required to achieve the necessary reducing atmosphere in a reasonable time can result in additional pumping equipment such as a booster or diffusion pump. This adds to system complexity, upfront cost, maintenance, and operating cost. Unfortunately, vacuum processes are often developed in, and organized around, single-chamber batch processing, so the actual purity requirement often gets distilled into an ultra-low vacuum level on the process specification. Consequently, these aggressive vacuum specifications are carried over to other types of equipment where they may not be necessary to achieve the same results.
Isolated Heat Advantage
Because the heat cage remains under vacuum throughout the process, there is less exposure to atmospheric contaminants. This allows oxidizing constituents to decay to very low levels leading to improved vacuum purity. Even though the absolute pressure is higher, the makeup of the remaining atmosphere is primarily inert. Given time for desorption to decay, it is entirely possible to have a purer environment at a higher pressure without requiring the complex pumping systems necessary in a single-chamber batch furnace. Reduction levels associated with diffusion pumping in single-chamber furnaces can be achieved at higher pressures with a two-stage or even single-stage pumping systems in an isolated heat furnace. This is one of the most overlooked and misunderstood advantages of isolated heat processing.
The Shift Toward Isolated Heat Furnaces
Despite the many challenges associated with single-chamber batch processing, the prevalence of these furnaces has remained high due to their simplicity and familiarity. So, why are multi-chamber furnaces gaining traction now?
“There is a pending perfect storm of market conditions poised to tip the scales.”
There is a pending perfect storm of market conditions poised to tip the scales. More and more traditional processes are shifting to vacuum for its long list of advantages, including tighter process control, flexibility, safety, insurance liability, and improved working environment, just to name a few. This push to convert more processes is driving a need to optimize efficiency and improve cost. The existing approach has known intrinsic inefficiencies and a limited growth path for improvement.
As more heat treaters either experience or compete with the benefits of multi-chamber isolated heat equipment, adoption will continue to accelerate.
Challenges and Considerations
While isolated heat furnaces offer numerous advantages, they are not without challenges. These systems are more complex, require a detailed specification process, and may not be suitable for very large components, intermittent operations, or applications requiring a high degree of flexibility. Many of the advantages of multi-chamber equipment show up in operating and maintenance costs. These benefits can be missed if these costs are not properly accounted for in the ROI analysis phase. Overemphasizing upfront costs can mean missing out on a much better return on investment for equipment with installation life in the range of 20–30 years.
Applications and Future Prospects
Isolated heat vacuum furnaces are not industry specific; rather, they offer advantages across a wide range of applications. Processes characterized by short cycle times benefit because a greater percentage of the floor-to-floor time is dead time and can be recovered, improving equipment utilization. Processes characterized by long cycle times benefit because they can be segmented and indexed at much faster rates, increasing throughput. Surface treatments can benefit from the process uniformity of smaller load sizes without sacrificing throughput. High-volume production environments, in particular, stand to gain the most. Whenever there is a need for more than one batch furnace or where there are numerous small parts in a large work zone, the efficiency and cost savings of continuous isolated heat furnaces truly stand out.
Conclusion
The industry’s focus on efficiency, reduced emissions, and lower operating costs makes isolated heat vacuum furnaces a promising direction for the future. While single-chamber furnaces will still have their place, isolated heat furnaces are becoming more prevalent for many heat treatment processes. Offering superior energy efficiency, better process control, and a more sustainable approach to thermal processing, these furnaces will enable manufacturers to provide high quality, cost-effective solutions that meet today’s market demands and future challenges.
About the Author:
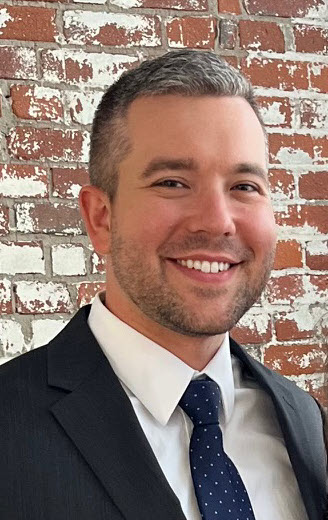
Product Development Manager
Gasbarre Thermal Processing Systems
Bryan Stern has been involved in the development of vacuum furnace systems for the past eight years and is passionate about technical education and bringing value to the end-user. Currently product development manager at Gasbarre Thermal Processing Systems, Bryan holds a B.S. in Mechanical Engineering from Georgia Institute of Technology and a B.A. in Natural Science from Covenant College. In addition to being a member of ASM, ASME, and a former committee member for NFPA, Bryan is a graduate of the MTI YES program and recognized in Heat Treat Today’s 40 Under 40 Class of 2020.
For more information: Contact Bryan at bstern@gasbarre.com.
Find heat treating products and services when you search on Heat Treat Buyers Guide.Com
Evolving Efficiency: Advantages of Multi-Chamber Isolated Heat Vacuum Furnaces Read More »