“Communication is key.” As heat treating equipment and processes evolve, it becomes critical that the accompanying control systems also develop to maintain “communication.” In this Technical Tuesday installment, guest columnist Stanley Rutkowski III, senior applications engineer at RoMan Manufacturing, Inc., discusses how digital control system communications have improved to increase energy efficiency for manufacturers with in-house heat treat operations.
This informative piece was first released in Heat Treat Today’s May 2024 Sustainability Heat Treat print edition.
Industrial furnace applications that rely on resistive heating will consume large amounts of electrical energy when processing their loads. Utilizing digital controls technologies to maximize this type of heating allows for a cleaner-and thus greener-approach to energy demands.
Typically, heat treat processes have a long duration (hours to days in length), and each load can have its own unique recipe in the amount of power required. With unique recipes, there tends to be a ramp-up phase (getting the vessel to temperature), followed by a soak phase (which demands more control over the power system), and then a cool-down phase (an even more controlled state). As the power is controlled through the furnace system, disturbances occur with different technologies. This starts with “tube technology,” then variable reactance transformer (VRT) technology, then silicon controlled rectifier (SCR) technology, and finally IGBT (insulated-gate bipolar transistor) technology. As these technologies have evolved, their ability to communicate information digitally has allowed for less disturbance in the power system and allowing both a less expensive energy bill and a cleaner energy usage for the process.
Definitions
Electrical Power
Power losses in an electrical system are defined by five aspects (Figure 1):
- Resistance (R): a function of the material cross section and the length of an electrical conductor.
- Reactance (XL): a function of the area in a circuit and is a vector 90 degrees offset from resistance.
- Capacitance (XC): a vector 180 degrees offset from reactance. In inductive circuits, capacitance can be added for power factor correction.
- Impedance (Z): the vector sum of resistance, reactance, and capacitance.
- Power Factor [cos(F)]: the ratio of resistance to impedance. In industrial applications, displacement power factor (DPF), the offset of the current to voltage waveforms, is used in the billing of electrical power.
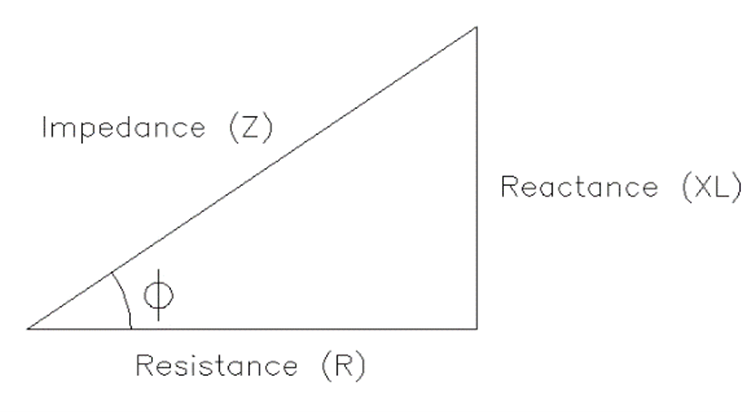

There are five unique aspects that define electrical power usage (Figure 2):
- Real power (kW): the amount of power that is generated.
- Reactive power (kVAR): the amount of power that is wasted.
- Total power (kVA): the rate at which power is consumed. This is also referred to as apparent power.
- Power factor (cos(F)): the ratio of real power to total power. In industrial applications, the displacement power factor (DPF) is the offset of the current to voltage waveforms and is used to bill for electrical power.
- Peak demand: the capacity required when the power grid experiences the highest power demand in a specified period of time.
3 Most Popular Types of Control Systems
For the most part, today’s furnace manufacturers use three main types of control systems: VRT, SCR, and IGBT. Each operates with slightly different methods to control how power goes into the heat treat furnace and creates heat.
VRT Control System
One traditional resistance heating setup uses a VRT control system that incorporates a saturable reactor, which controls the power applied to the transformer in the system (Figure 3). The control transformer on the output side of the transformer feeds back to the reactor to set the limit on the input power to the transformer.
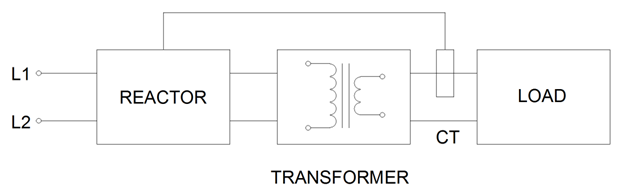
SCR Control System
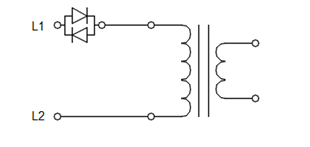
Another traditional resistance heating setup uses an SCR control system that includes dual thyristors (gated diodes) to control the amount of power applied to the primary of a transformer.
The SCR control delays the start of the waveform, and the control point is reset when the waveform crosses the zero line.
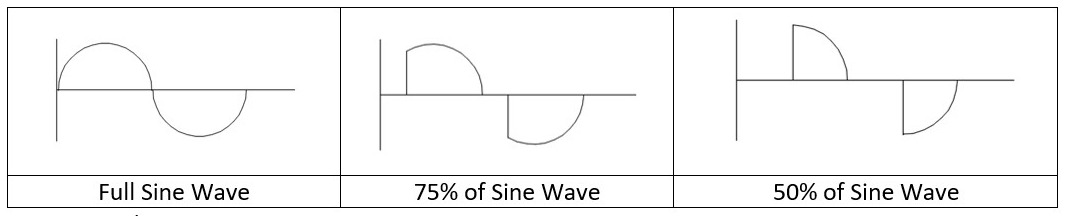
IGBT Control System
Finally, an IGBT control system uses a diode bridge, capacitor, and switching transistors to control the amount of power applied to the primary (i.e., main power input of a transformer). The input frequency to the transformer is controlled by the switching transistors. Since the IGBT control system utilizes all three phases of the power system, the IGBT control can be set to a particular phase for the zero cross (for phase orientation in the application, synchronous mode) or left floating (non-synchronous mode), as is demonstrated in Figure 6. The input voltage to the transformer is increased by the operation of the IGBT control. As such, potential energy savings may be had with these types of controls as compared to tradition controls (such as on-off contractors, time proportioning controls, or other types of current proportioning control systems).
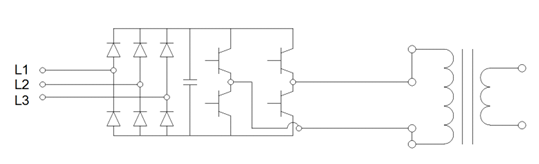
Synchronization with the IGBT can be to the incoming lines (A, B, or C phase) and can be offset from each of the phases. The ability to offset from a phase allows for traditional arrangements (Single Phase, Scott-T, Delta and Wye) as well as unique offsets allowing for additional vector heating in the application with AC outputs. The unique arrangements beyond the traditional systems could allow for more uniform heating of the part and less energy being consumed during the process.
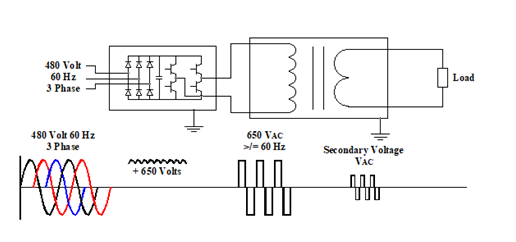

Advantages of Utilizing Communications
As technology for controlling heating systems has evolved, and with an emphasis on clean energy sources, the ability to communicate with the control system has increased as well. This communication allows for more precise control of the run for the load, improved power usage (better power factors and less peak power usage as well as less total power usage), and inputs into a preventive maintenance program.
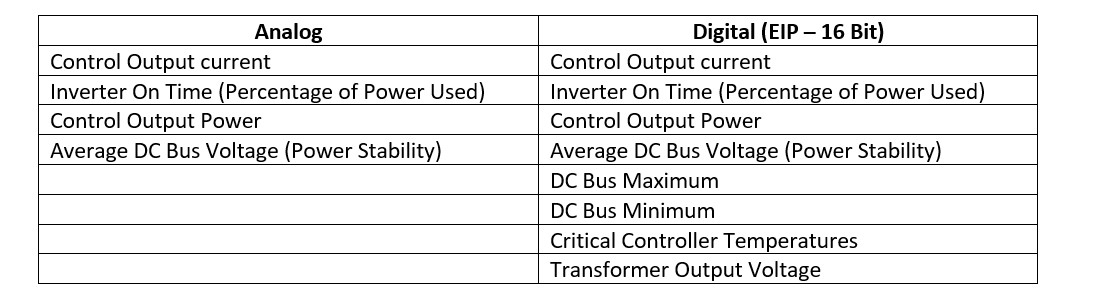
With an IGBT system, both analogue and digital control communications are available today. See Table A for a comparison on how each control option works.
In addition to the EIP defined pieces, there is the ability to access the FPGA system for graphical outputs that can be downloaded into another system in your process for storage, comparisons, or general record keeping for a part run. The FPGA is an internal processor in the control that allows for more data, charting, and diagnostics to be captured and used by the system for both energy consumption and possible preventative maintenance purposes.
Why does this matter? Let’s turn to some possible ways of using the data generated from digital controls systems:
- Evaluate average, minimum, and maximum DC bus voltages to plan for the best time and day to run heat treat jobs. For high power draw jobs, planning ahead can minimize power costs; similarly, knowing power trends can be helpful to plan jobs requiring sensitive control of the heating.
- Evaluate transformer output voltage to allow the system to detect any shorts in the process. If the controller output and transformer output diverge from the known turns ratio, a change has occurred in the system. This could be corroborated if controller on time and output power do not trend.
- Track furnace run records with EIP communications and FPGA data. This will be most helpful in processing lots of data, as is the case for Milspec records.
- Evaluate changes in power factor to monitor any loose cables, and so avoid reactive power losses.
- Evaluate the current versus the voltage to monitor the resistance of the system. If there is an increase in the resistance, you could project the trends in wear of the heating elements, therefore predicting future required maintenance.
- Evaluate the critical control temperatures of the system to know if it is being run close to, or above, its ratings or if there is a disturbance in the cooling systems.
- Use knowledge of power usages and power stability to update recipes for load runs so they use less power over the total run; this allows for a less costly power-savings solution. With less power usage, more output of the total facility can be had as each station contributes less to energy consumption
Even more benefits can be realized when users and builders of furnace systems and component manufacturers collaborate in the design of the total system. Such dialogues lead to the creation of more interactive and intuitive solutions that minimize power consumption, minimize downtime, and maximize outputs. These practical benefits are the foundation of a greener system.
About the Author:
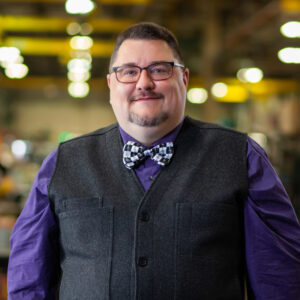
Senior Applications Engineer
RoMan Manufacturing, Inc.
Stanley F. Rutkowski III is the senior applications engineer at RoMan Manufacturing, Inc., working on electrical energy savings in resistance heating applications. Stanley has worked at the company for 33 years with experience in welding, glass and furnace industries from R&D, design, and application standpoints. For more than 15 years, his focus has been on energy savings applications in industrial heating applications.
For more information: Contact Stanley at srutkowski@romanmfg.com.