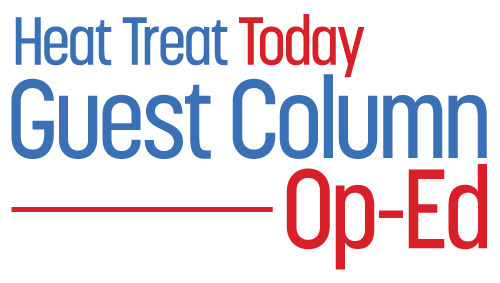
Heat treating aluminum presents a unique concern due to the operating conditions of high temperature, chemical corrosion, mechanical abrasion, and temperature variation. Guest columnist Roger M. Smith, director of technical services at Plibrico Company, LLC, examines the critical role the refractory lining plays in the success of manufacturing aluminum, why a refractory is susceptible to cracking under extreme conditions, and how to select and prepare refractory linings to achieve a longer service life.
This informative piece was first released in Heat Treat Today’s August 2024 Automotive print edition.
A significant concern when manufacturing aluminum metal is the practical service life of the furnace. The service life is driven by the refractory lining’s ability to resist the various operating conditions within the furnace, such as high temperature, temperature variation, chemical corrosion, and mechanical abrasion. Ideally, a single refractory composition would be capable of withstanding all these conditions and readily available at a low price.
Unfortunately, this is rarely the case.
Proper refractory selection is often about finding the best balance between price, properties, and performance for the given application and operating conditions. A refractory capable of high strength and abrasion resistance is often susceptible to cracking caused by extreme temperature variations, commonly referred to as thermal shock. However, a material capable of withstanding thermal shock without catastrophic cracking may be vulnerable
to chemical corrosion. Finding the best balance of material properties for each zone in each furnace is important for maximizing the service life of a furnace.
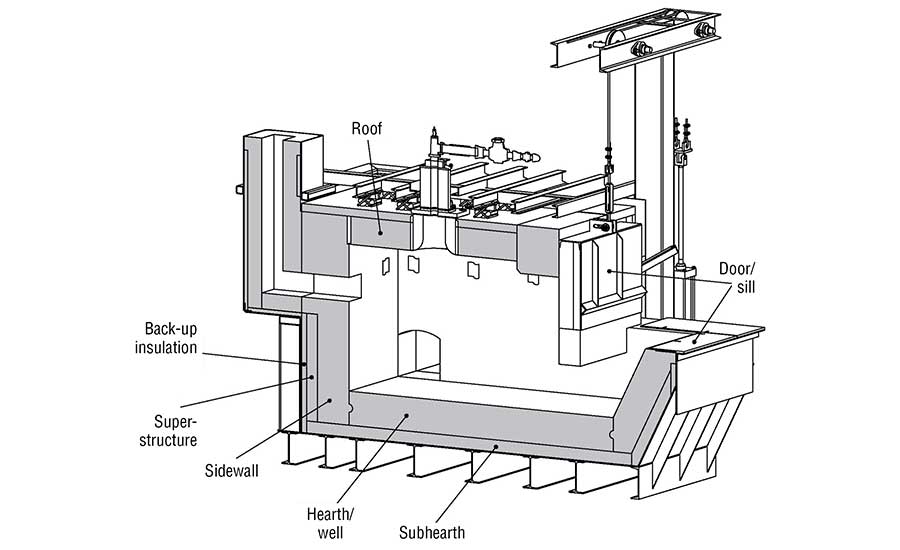
Refractory Under Attack — Requirements for Melting Aluminum
The refractory lining in an aluminum furnace (Figure 1) must endure various chemical reactions that occur while the furnace is in operation. There are three separate regions to consider: above, below, and at the melt line. Above the melt line, the refractory must withstand attack from various alkali vapors. Alkali vapors can be produced from flux used in the aluminum and from the combustion products used to heat the furnace. Below the melt line, the refractory must withstand molten aluminum. At the melt line, the region commonly referred to as the bellyband area, there is a triple point where the refractory, atmosphere, and aluminum interact.
The refractory below the melt line comes in direct contact with liquid aluminum when the furnace is in operation. This contact can create a chemical reaction zone where oxides on the surface of the refractory can be reduced, such as silica (SiO2) to form silicon. Conversely, aluminum can penetrate into the refractory lining either through the same redox reactions or through infiltration due to capillary forces.
Aluminum forms corundum (Al2O3) when it oxidizes. This results in a change of the crystal structure from face-centered cubic to hexagonal, which causes a significant volume expansion. When corundum is formed inside the refractory lining, the change in volume creates cracks, which lead to more infiltration and more cracks until the refractory lining ultimately fails.
Wetting the Refractory
One method for reducing the reaction zone is to prevent the aluminum from “wetting” the refractory (see Figure 2). A liquid’s ability to “wet” a surface is defined by the contact angle of the liquid. When the contact angle between the liquid and the surface is greater than 90 degrees, then the liquid is said to wet the surface. When the contact angle is less than 90 degrees, the liquid does not wet the surface. A liquid that does not wet the surface is analogous to water beading on a car that has been freshly waxed. When aluminum does not wet a refractory, it is not able to react with the refractory and is not able to penetrate the lining.
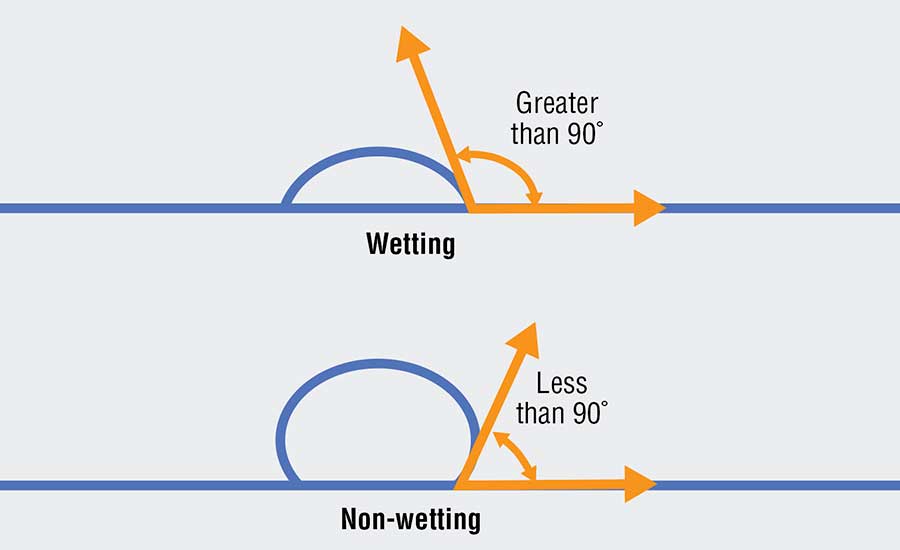
Various additives can be used to reduce aluminum’s tendency to wet a refractory. Some of the most used additives include barium, boron, or fluoride. They modify the surface chemistry of the refractory and reduce aluminum’s ability to react and penetrate. Using additives such as these greatly extends the effective service life of a refractory lining.
While non-wetting additives can be beneficial to extending the service life in areas where there is contact with molten aluminum, there are no benefits when not in aluminum contact. They do not protect from alkali attacks above the melt line. They do not enhance the abrasion resistance of the material. They do not improve the thermal shock resistance of the material. Furthermore, these additives are volatile. When exposed to temperatures above 1700°F (927°C), they begin to lose their effectiveness because they chemically react with other materials in the refractory and change. The additives can also be costly, which raises the price of the refractory compared to one with the same composition but without the additive.
The presence of non-wetting additives can have some negative effects on a refractory. Tests have shown that a 1% addition of a fluoride additive in a conventional castable can reduce the hot modulus of rupture (HMOR) by as much as 30% at 2000°F (1093°C). The effect can be even more significant in a low-cement castable. The loss in hot strength is likely attributed to the formation of a glassy phase induced by the additive. Fluoride and boron are both well-known glass formers and will form a glassy phase at the grain boundaries at high temperatures, which reduces the bond strength between individual grains and the overall strength of the bulk material.
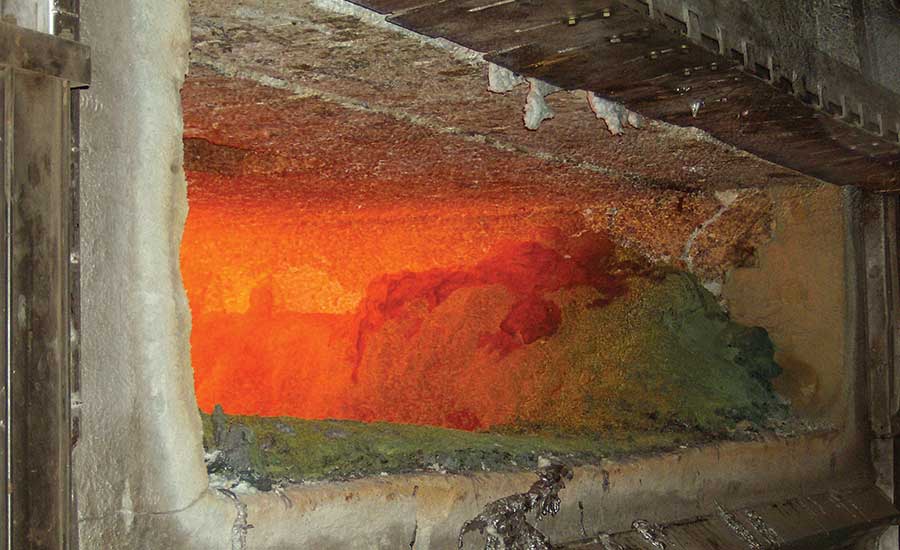
Balancing Refractory Properties
The advantages and disadvantages of a refractory material should be considered when selecting materials for an aluminum furnace. The sidewalls of a furnace all come in direct contact with molten aluminum.
The upper sidewalls must be scraped to remove aluminum that splashes up to prevent corundum growth. The refractory selected for its sidewalls should be abrasion resistant to protect from mechanical scraping and non-wetting to protect from corundum growth. The hearth and well are submerged in aluminum, but they do not see the same level of abrasion as the sidewalls. The sub-hearth may see some molten aluminum but must also provide support, so a strong, non-wetting refractory should be used.
The door and sill will experience temperature fluctuations every time the door is opened, and they will be exposed to abrasion as the furnace is charged. Materials that are resistant to thermal shock and abrasion should be selected. The roof and superstructure need to be strong and resistant to alkali vapors. Backup insulation should be selected to reduce heat loss, but it should be of a composition that has moderate resistance to molten aluminum in case of refractory failure at the hot face.
In all these zones, the operating conditions of the specific furnace must be considered, and the balance of properties must be adjusted case-by-case. The primary failure modes must be identified, and materials should then be adjusted accordingly.
The Key to Refractory Selection
The operating conditions in an aluminum furnace require a refractory lining with different benefits in different zones. At the furnace door, the refractory can experience drastic fluctuations in temperature that can cause cracking. The upper sidewalls will develop scale that has to be scraped off, so the refractory needs to be abrasion resistant.
The lower sidewalls come in direct contact with molten aluminum and need to resist chemical attacks and aluminum penetration to avoid corundum growth. Finding a cost-effective refractory that can meet all these requirements is very difficult, but it can be done with sufficient research. Careful material selection that considers the needs and operating conditions of a particular furnace is important for maximizing the service life of a refractory lining.
About the Author:

Director of Technical Services
Plibrico Company, LLC
Source: Plibrico
Roger Smith is a seasoned professional in the refractory industry. With a master’s degree in Ceramic Engineering from the University of Missouri – Rolla, Roger has over 15 years of experience in the processing, development, and quality assurance of both traditional and advanced ceramics. He has a proven track record in developing innovative ceramic formulations, scaling up processes for commercial production, and optimizing manufacturing operations.
For more information: Visit www.plibrico.com.
This article was initially published in Industrial Heating. All content here presented is original from the author.