“Many metallurgists or heat treat engineers only think in terms of water or oil for quenching steel. Water is the most common quench medium, followed by oil. However, polymer quenchants have made significant inroads into these traditional choices…”
In today’s Technical Tuesday feature, Greg Steiger and Keisuke Kuroda of Idemitsu Lubricants America share an original content article on the composition and uses of polymer quenchants, specifically polyalkylene glycol.
Introduction
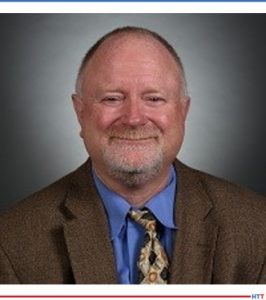
Senior Key Account Manager
Idemitsu Lubricants America
Many metallurgists or heat treat engineers only think in terms of water or oil for quenching steel. Water is the most common quench medium, followed by oil. However, polymer quenchants have made significant inroads into these traditional choices.
The advantages of water are abundance, low cost, lack of flammability, and the ability to achieve high hardness. Still, there are many disadvantages associated with water as well. These are all associated with the very aggressive quench obtained from water. Issues such as quench cracking, distortion and soft spots from uneven cooling are just a few of the drawbacks of water.
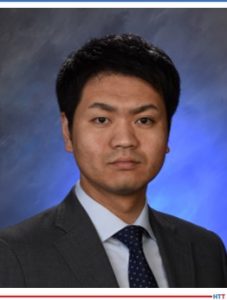
Technical Advisor
Idemitsu Lubricants America
Oil quenchants do not offer the hardenability of a water quench because the quench speeds of oil are more limited than those of water. Quench oils also pose a fire hazard which can create workplace environmental issues such as smoke generated during the quench process. Additionally, the disposal costs of used quench oils continue to increase as time goes on. Limited options for applications requiring a quench speed between oil and water were available until water soluble polymers were introduced to the market in the mid-20th century.
With water soluble polymers heat treaters could vary the concentration in water to achieve oil like quench speeds. Furthermore, using warm or hot water provided the ability to increase the quench speed to approach that of water yet minimize the quench cracks and distortion due to the high quench severity of oils.
Historically, polymer quenchants were used in hardening steel and in nonferrous (aluminum) applications and continues to be a popular choice for these operations today. However, its use in induction hardening has grown exponentially, and as such, polymer quenchants have become much more important to modern manufacturing and heat treating.
1. Types of polymer quenchants
Today, there are many different types of polymers in use. Examples of these types of polymers include polyacrylates, polyvinyl alcohol, polyvinylpyrrolidone, polyethyloxazoline, polyethylene glycol and the most popular polyalkylene glycol (or PAG). The types of polymers and their characteristics are seen below in Table #1.
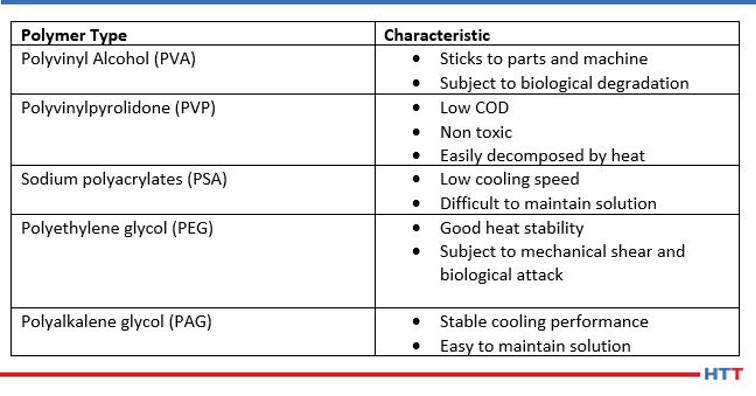
While each of the chemistries listed in Table #1 are in use today, the scope of this paper will be limited to the most used chemistry, polyalkylene glycol.
1.1 Polyalkylene glycols and inverse solubility
A polymer quenchant is composed of more than just the water-soluble polymer. In typical polyalkylene glycol polymer quenchants, water makes up the largest ingredient. However, there are additives such as ferrous corrosion inhibitors, nonferrous stain and oxidation inhibitors, alkalinity buffers, defoaming agent, biocides along with the polyalkylene glycol in typical polyalkylene glycol quenchants. Chemically, a polyalkylene glycol consists of nothing more than carbon, hydrogen, and oxygen. The chemical structure for a polyalkylene glycol is seen in Figure #1. The m and n represent the number of molecules contained in the polymer. The higher the values of m and n, the thicker and more viscous the polymer becomes.
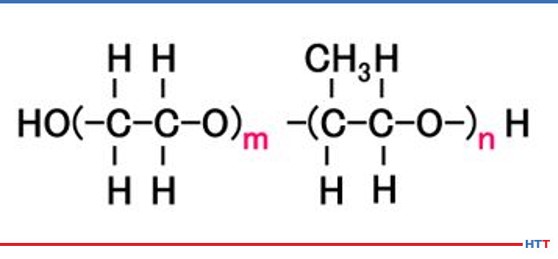
In examining the chemical structure of a polyalkylene glycol it can be seen there or OH and H molecules on each end of the polymer. As we learned in high school science classes, like dissolves like. Water is composed of these same compounds and this is why the polymer is soluble in water. However, a polyalkylene glycol exhibits inverse solubility at higher temperatures due to a phenomenon called a cloud point. At 70°C (approximately 160°F) the polyalkylene polymer becomes insoluble in water. By being no longer soluble in water the polymer then coats the part being quenched and controls the cooling rate to provide a slower quench speed than pure water thereby reducing or eliminating the risk of quench cracking and distortion. A demonstration of the cloud point phenomena is shown in Figure #2.
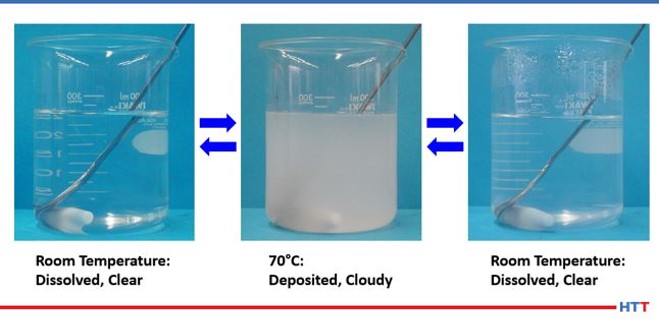
In examining cooling curves generated using the test method JIS K2242-B Heat Treating Fluids cooling curves for plain water and c solution can be examined. Using the cooling curves shown in Figure 3 the cooling curve for the water is on the left and the cooling curve for the polyalkylene glycol (PAG) is on the right. As cooling curves are shifted to the right the quench severity and quench speed both decrease. The inset shows a simulation of how a polyalkylene glycol polymer exhibits inverse solubility at elevated temperatures and coats the part being quenched to control the cooling speed.
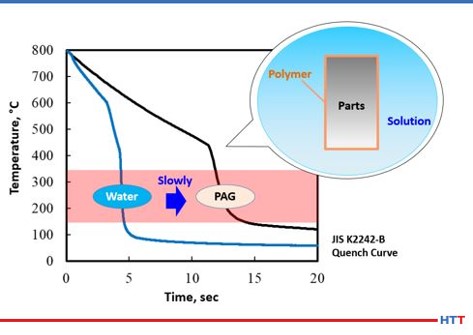
One of the unique properties a polyalkylene glycol possess that a quench oil does not is the ability to vary the cooling rate of the solution by concentration. Unlike an oil, a polyalkylene glycol solution is diluted with water and the amount of polymer to control the cooling rate varies with concentration. For instance, a 10% concentration of a polyalkylene glycol solution will have a faster and more severe quench rate compared to a 30% solution of the same polyalkylene glycol. Figure #4 shows a comparison of cooling speeds of various polyalkylene glycol solutions versus pure water.
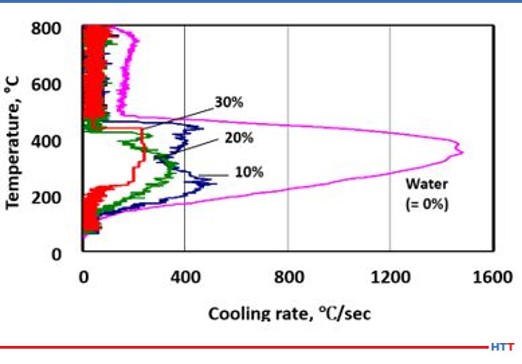
2. The deterioration of a polyalkylene glycol polymer
While modern polyalkylene glycol quenchants are formulated to provide excellent corrosion and biological protection. The simple act of using them to quench parts creates conditions where the polymer deteriorates. As stated above, it the function polymer becomes inversely soluble at elevated temperatures and coat the parts to control cooling. This will also cause the depletion of the polymer and other additives through drag out. Similarly, as hot parts come into contact with the polymer, pyrolysis occurs. As a result of pumping, the polymer solution the polymer is mechanically sheared.
The solution undergoes mechanical shearing when a solution is continually circulated through a system by using a mechanical pump. The less viscous the fluid the less susceptible the fluid is to mechanical shearing. Table 2 shows the viscosity of three widely available commercial polyalkylene glycol polymers.
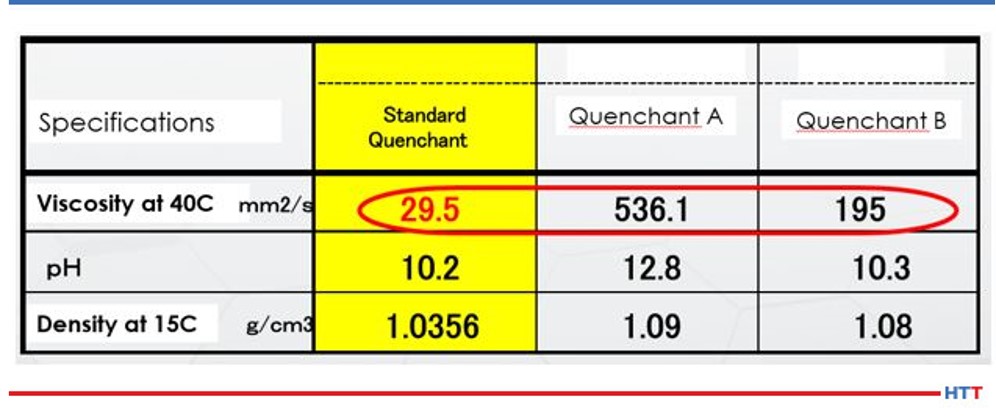
Table 2 shows that Quenchant A is over 18 times greater than the viscosity of the viscosity of the standard quenchant, and Quenchant B is over 6 times the viscosity of the standard quenchant. Noting change in viscosity makes it is easy to see how mechanical shearing can affect polymers in different ways. As the solution is sheared and loses viscosity, the cooling properties of the polymer also change. Simple physics shows that the heat transfer properties of a thin, less viscous fluid, such as water, dissipates heat better than a thick, viscous fluid such as maple syrup.
In addition to mechanical shearing reducing the viscosity of the polymer, pyrolysis also creates a similar breakdown in the polymer. Pyrolysis is a chemical process where the polymer becomes thinner and less viscous due to the long chain length polymer being thermally broken into less viscous shorter chain polymers at high temperatures. Figure #5 shows the effects of mechanical shearing and pyrolysis on a short chain, less viscous standard quenchant polymer.
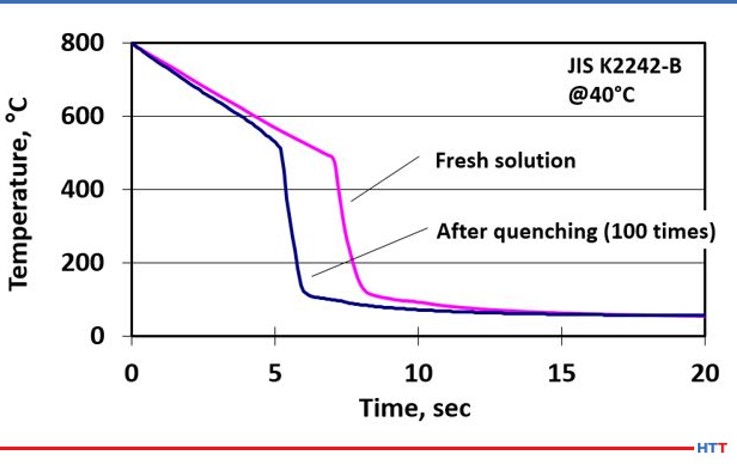
The severity of how pyrolysis and shearing affect the quench as the cooling speed of the polymer quenchant has clearly increased. This increase in the cooling speed is shown as the curve has shifted to the left. The increase in cooling speed and quench severity are directly related to the thinning polymer viscosity, which is directly attributable to mechanical shear and pyrolysis. To further emphasize this point, let’s look at how users of polyalkylene glycol quenchants determine concentration.
A handheld refractometer is typically used to measure what is often referred to as the refractometer reading. Some users and suppliers of polymer quenches instead use the proper term Brix%. The Brix% measures the amount of polymer dissolved in water and the contaminants within the polymer tank. Contaminants can be thought of as anything dissolved or emulsified in water. Several examples of dissolvable materials include hard water minerals such as calcium, or magnesium as well as any water soluble coolants or rust preventatives used in machining prior to heat treating. Some emulsified oils can be common machine oils, like hydraulic oil, that have leaked into the polymer tank.
Because all these dissolved or emulsified materials can impact the concentration levels of the polymer, most suppliers will ask for a periodic check of the solution be done using a benchtop refractometer. This reading measures how much light passing through a prism is refracted or bent by the polymer. Because the dissolved contaminants do not refract the light this is a more accurate method of determining the polymer concentration. However, it is a lab based piece of equipment and is not portable and must be liquid cooled to 20°C (68°F). Therefore, the portable Brix meter is typically preferred in heat treating operations.
The most preventable form of deterioration of a polymer quench is from contamination by tramp oils, bacteria and in severe cases mold. Tramp oils are oils in the fluid that are not formulated into the quenchant. Because a polyalkylene glycol polymer does not contain oil any oil in the solution it is considered to be tramp oil. Regarding bacteria, there are two basic types: aerobic and anaerobic. Aerobic bacteria can live in the presence of oxygen and anaerobic bacteria thrive in oxygen depleted environments. The goal for users of polymer quench is not to eliminate bacteria entirely. This is because we do not live in a sterile environment. The water we drink, food we eat, and the air we breathe all contain bacteria. Instead, the goal of polymer quenchant suppliers and users is to prevent anaerobic bacteria and its “Monday morning odors.” Figure #6 shows a mockup of a typical sump containing a polymer quenchant and various contaminants.
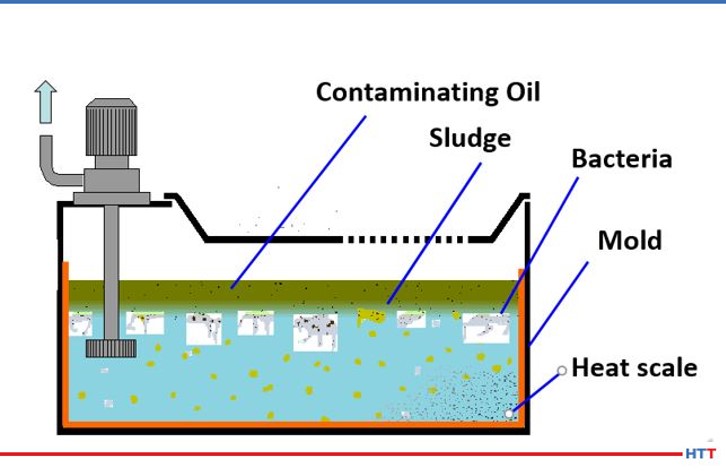
Above, the sludge layer consists of a mixture of tramp oil and polymer that has not gone back into solution. The most likely source of the tramp oil is from hydraulic oil or other machine oil leaks. This layer creates an impermeable layer against oxygen, leading to anaerobic bacterial growth. The tramp oil layer may be removed using an effective tramp oil skimmer. The anaerobic bacteria produce the rotten egg smell of hydrogen sulfide. The solution to eliminating the anaerobic bacteria is very simple. The removal of the tramp oil layer will allow oxygen to permeate through the solution through normal usage. However, removing the tramp oil layer is not enough. The second portion of the sludge layer is the polyalkylene glycol that emulsified with the tramp oil. Removing the tramp oil will cause this heavier than water polymer to sink to the bottom of the tank. This heavy polymer will prevent oxygen from reaching the material below the polymer once again creating a zone of anaerobic bacterial growth. The solution here is to use a shorter chain, less viscous polymer that will require less agitation to resolubilize in water at lower temperatures.
The effects on cooling speed are seen when a fresh solution of polymer quench is compared to the cooling speed of the same fresh polymer solution when a small amount of emulsified tramp oil and polymer is added to the same fresh polymer solution. This results in a shift of the cooling curve to the right, which slows the overall cooling speed and can result in lower case depth and softer than expected hardness results. The cooling curve is seen Figure #7.
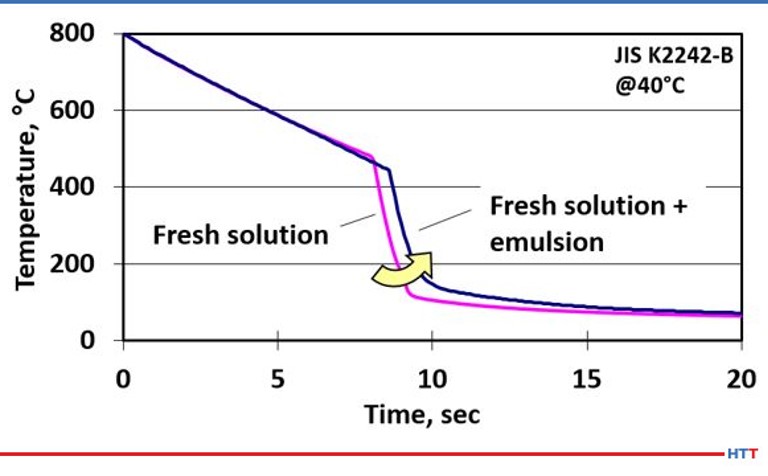
Another very common source of polymer deterioration is by contamination of heat scale which can easily be removed via filtration. Most individual induction hardening machines use an internal filter media bed. The micron size of these media filters can vary from the small ~2-3 micron to the large ~50 micron. For larger central systems and through hardening furnaces a canister filtration system is typically used. The micron size of the filtration media is typically an economic decision as the smaller pore size increases the cost of the filter. Also, the smaller the pore size the quicker the media will blind. A happy medium between cleanliness of the polymer solution and economics is typically found between 10- and 25-micron filter media.
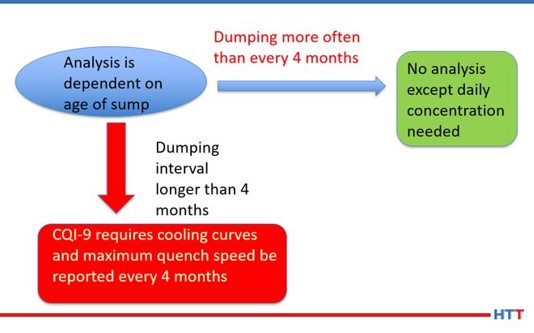
While CQI-9 requires only a daily concentration check and a cooling curve analysis for systems over four-months old, many suppliers of polymer quenchants recommend additional tests such as pH, viscosity, refractive index and other testing that is not practical for users of polymer quenchants to perform. Table #3 lists the test and frequency of the suggested test for a polymer quench solution.
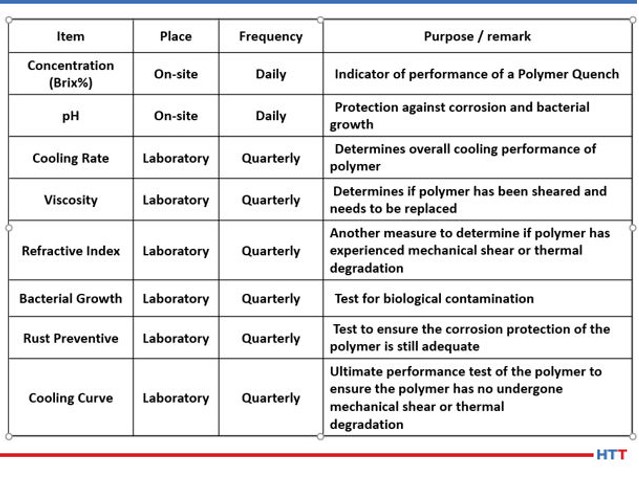
3. CQI-9 testing
This section will describe the testing required under CQI-9 as well as the frequencies and the reasons behind the suggested periodic tests.
As mentioned earlier in this paper a daily concentration check is needed for a polymer solution. The most convenient and easiest method is to use a handheld refractometer. The operation of the handheld refractometer is seen in Figure #9.
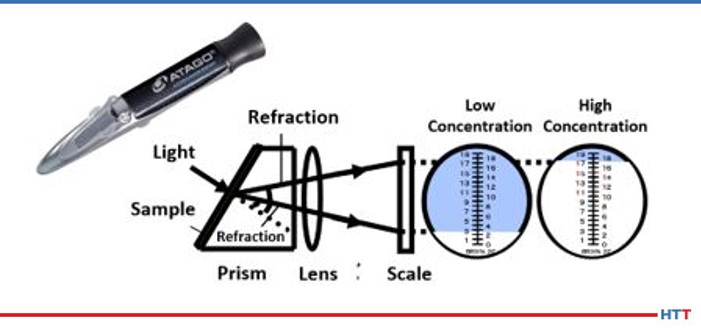
As previously noted, the mechanical shearing and effects of pyrolysis on a polymer are a reduction in the viscosity of the polymer in solution. Additionally, these same effects change the cooling properties of the polymer, as seen in Figure #6; the shifting of the cooling curve only describes the overall cooling curve of the polymer solution.
However, CQI-9 requires a cooling curve analysis. As a part of a compete cooling curve analysis, the cooling rate of the polymer should also be determined. Because there is a direct relationship between viscosity and cooling rate, it follows that as the effects of mechanical shearing and pyrolysis reduce the viscosity of the polymer in solution the cooling speed of the polymer will also increase as shown in Figure #101
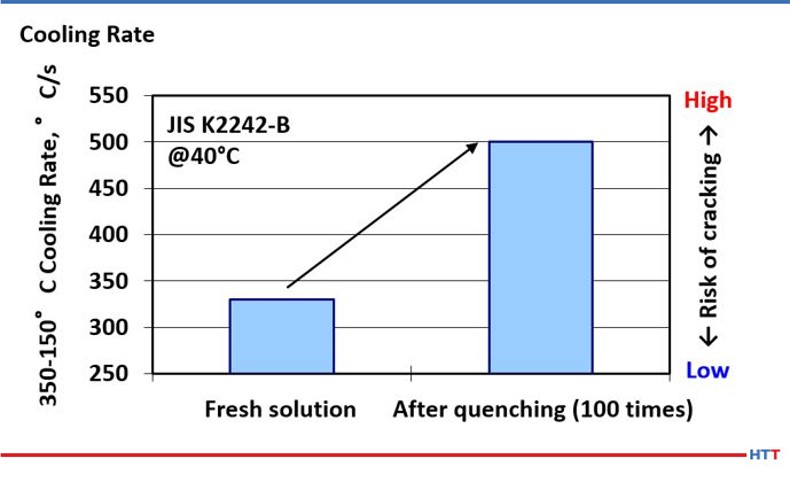
Knowing the pH of a solution is imperative for a few reasons. The higher the pH the higher the alkalinity and the better the protection against bacterial attack. Alkalinity is a measure of protection against corrosion. However, having too high of a pH can result in skin irritation. In Figure 11 below, the reader can see what pH manufacturers of polymer quenchants recommend.
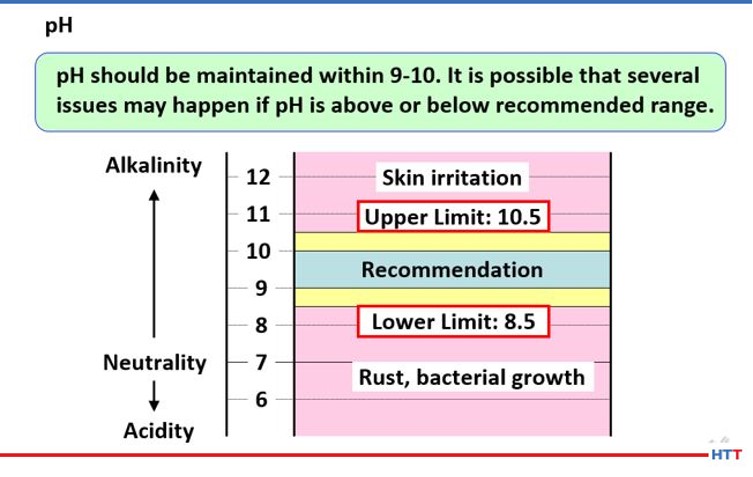
To run the bacterial testing on a polymer solution requires a special media called an agar to grow the bacteria colonies. These aerobic colonies are measured as a power of 10. Typically, these colonies are measured in the range of >100 to 10(7). In rare cases yeast and mold may also grow in a polymer quenchant. Once again, the colonies are measured in powers of 10. The typical range is >10 to 10(5). Figure #12 shows a pictogram of each level of bacterial and yeast and mold contamination. It is best to let the polymer supplier run this testing since it is dependent on sample handling and testing at a specified constant.
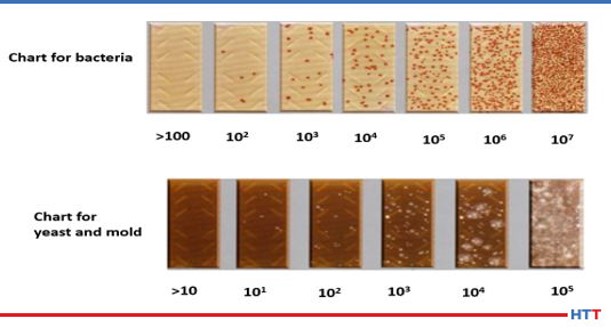
The last piece of maintenance to be addressed in this paper is the proper mixing of a polymer. Water should be added to the tank first. Once the water level reaches approximately ¾ of the full level, the water additions can end. The next step is to agitate the water while slowly adding in the polymer. It is important that the polymer not be added before the water as the polymer is much denser than the water. This will cause the water to remain on top of the polymer and will result in incomplete mixing. Once the polymer has been completely mixed into the water, a handheld refractometer can be used to determine the concentration, and then any needed water or polymer additions can be made.
Conclusion
This paper showed that the ability of a polyalkylene glycol to effectively quench and harden carbon steels is determined by a variety of factors:
- Concentration
- Polymer chain length
- Viscosity of polymer
- Mechanical shearing
- Pyrolysis
- Age of the polymer quenchant
The cooling speed of a polymer quenchant by concentration can be seen in Figure #4. The cooling speed varies by concentration because the amount of water present in the solution varies. The less dense water dissipates the heat faster than ticker denser polymer. Figure #13 shows the cooling curves of Quenchant A and the standard quenchant at concentrations of 10%, 20% and 30%. In Figure #13 the reader will notice less variation in the cooling curves for the standard quenchant compared to Quenchant A. This is due to the major differences in viscosity of the two products shown in Table #2.
Mechanical shearing will affect the cooling rate of a polymer by causing the viscosity of a thick polymer to thin out and become less viscous. Figure #14 shows how selecting a polymer with a polymer with a lower viscosity that is less resistant to mechanical shear and pyrolysis will exhibit less change in the cooling rate after continuous quenching.
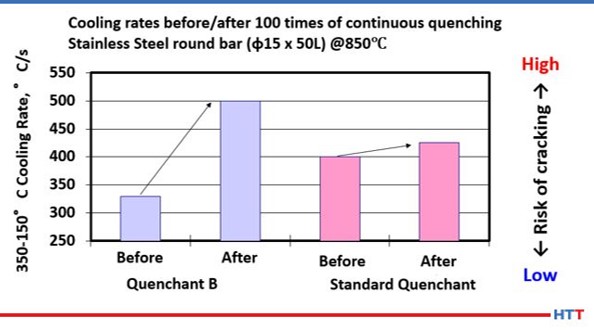
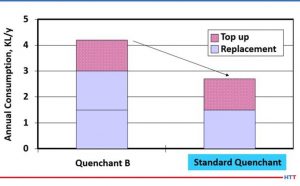
In summary, a less viscous polymer is preferable due to the consistency of the quench, cooling speeds, and longer sump life than a more viscous polymer. Additionally, it will require less agitation to remix with water once the temperature of the solution is below the inverse solubility temperature of the polymer. Because the polymer remixes easily with water it does not plate out on the machines and fixtures and the carryout on the parts is greatly reduced. Since there is less plate out on the fixtures and machines along with the polymer remixing with water, there is a reduced need to dump the machine sump due to house cleaning issues. When the polymer goes back into solution, it does not settle to the bottom of the tank where it can create an environment for anaerobic bacteria growth as well. Figure #14 shows the annual volume reduction experienced when an actual customer switched to a lower viscosity polymer which resulted in a longer sump life and less drag out.
REFERENCES:
1. K. Kuroda, G. Steiger. The Importance and the Proper Way to Monitor Polymer Quenches. 2020 Furnaces North America. (All figures and tables are taken from this source.)
About the Authors: Greg Steiger is the sr. key account manager of Idemitsu Lubricants America. Previously, Steiger served in a variety of research and development, technical service, and sales marketing roles for Chemtool, Inc., Witco Chemical Corporation, D.A. Stuart, and Safety-Kleen. He obtained a BSc in chemistry from the University of Illinois at Chicago and is currently pursuing a master’s degree in materials engineering at Auburn University. He is also a member of ASM.
Keisuke Kuroda is the technical advisor for a line of industrial products which includes quench products for Idemitsu Lubricants America. Before joining Idemitsu in 2013, Keisuke held various sales and marketing positions. Keisuke holds a master’s degree in physics from Kobe University.